Molokai student Mallory Go has co-authored a paper published in The Astronomical Journal under the title “Magnetic Fields in the Horsehead Nebula using data from the James Clerk Maxwell Telescope. Go (who graduated in 2021) was awarded time under the Maunakea Scholars program – a program that gives students at High Schools in Hawaii access to the telescopes on Maunakea.
Using the James Clerk Maxwell Telescope (JCMT) in 2018 Go obtained unique images of the Horsehead Nebula in polarized light – a technique astronomers use to reveal the magnetic field within the Nebula. Despite the Horsehead Nebula being such an iconic cloud, which is famous amongst astronomers, Go was the first to propose such observations.
“When I heard about the Maunakea Scholars program I was excited. It seemed to me to be such a fantastic opportunity to use the Telescopes on Maunakea” said Go reflecting on her experience “I chose to study the Hosrehead nebula because I thought it was beautiful and I didn’t find much research on it.” Once the proposed observations were taken, and working with astronomer Dr Parsons, Go presented her work as part of her Science Fair representing Molokai`i High School. After this, the data were shared with top astronomers in the field who perused the work further. Jihye Hwang, Kate Pattle and Jongsoo Kim built on Go’s observations to perform a quantitative analysis of the strength and role of magnetic fields in the region.
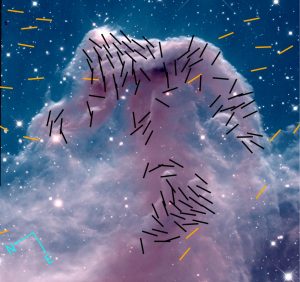
Magnetic field detections overlaid on a two-color composite of Hubble Space Telescope image taken at two near-IR wavelengths (Mikulski Archive for Space Telescopes). Black and orange segments show magnetic field orientations inferred from JCMT and Palomar Observatory. Credit: Hwang et al. 2023.
“The data are impressive and what they tell us is even more impressive,” said co-author Dr Kate Pattle, from University College London (UCL), UK. “I am delighted that Mallory has given us the chance to work on such a beautiful and iconic region of the sky – and what we’ve found helps us to understand why the Horsehead Nebula has the shape that it does. These observations tell us a story of two dense regions hidden in the Horsehead. We see a ridge of warm gas and dust – the head and mane of the horse – that is interacting with the ultraviolet photons from nearby bright young stars. But sheltered behind that ridge, we see a cold clump of dense material which we think will go on to form a new solar system like our own. What’s so new and exciting about these observations is that we get to see for the first time what the magnetic field within these regions is doing.”
The observations obtained by Go in 2018 were taken by one of the JCMT’s most cutting-edge instruments: POL-2. POL-2 is a polarimeter which is able to take measurements of the alignment of interstellar dust that can be influenced by magnetic fields in space. “You can think of POL-2 as a pair of polarized sunglasses sitting in front of the telescope,” said Head of Operations Dr Harriet Parsons. “In Hawaii many of us are used to wearing polarized sunglasses – they help us to see better by cutting down glare – but at the telescope by rotating the polarized lenses we analyze the brightness of the light being observed and deduce if it is under the effect of magnetic fields. Astronomers can look at clouds of gas and dust using such instruments – regions both within our own galaxy or beyond – and expand their understanding of what shapes them.” In addition the published paper makes use of additional data observed with two of the other instruments available at the JCMT.
Commenting further on this data Dr Pattle said “The JCMT is a fantastic tool: we have used data from three of the telescope’s instruments to measure how bright and how dense the region is, and what its gas and magnetic fields are doing. We see that the interaction between the head and mane of the horse and the nearby young stars has significantly reordered the magnetic field – we suggest that the magnetic field has been folded back on itself along our line of sight as the Horsehead formed. Interestingly, though, the magnetic field in the cold clump sheltered by the ridge seems not to have been affected by the interaction that created the Horsehead – it behaves exactly as we would expect magnetic fields in an isolated dense clump to do. This supports the theory that the dense clump is sheltered by the ridge. This gives us important insight into how stars can continue to form even in regions like the Horsehead, where the cold gas that provides the material for new stars is being eroded by photons from nearby young and hot stars. We expect that our own Sun formed as part of a cluster of stars, and so looking at how stars form in the Horsehead Nebula may give us an insight into our own Solar System’s past.”
As a participant of the Maunakea Scholars program Go understands that some people might be surprised to find that she is now a student at Brown University studying Public Health. Commenting on this Go said “even back in 2018 I knew I would go to college to major in Public Health but I joined the Maunakea Scholars program because it sparked my interest. That’s a personal philosophy of mine; pursue the things that interest you.”
Reflecting on the publication of the data taken back in 2018 Go said “it’s wild to see that this work is now published for other astronomers to build on. That region is even more beautiful to me now I know this story of the two clumps with subtly different stories hidden within.” And for her current career path “I love Brown University and I am really enjoying studying Public Health, I’m the Class of 2025 but I might also look at doing post-graduate studies here.”
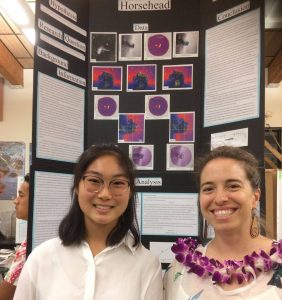
Mallory Go (left) presenting her work on the Horsehead Nebula (right: JCMT astronomer Dr Harriet Parsons) at the Molokai High School Science Fair 2019.
Additional information
The Research
This research was published under the title “Magnetic fields in the Horsehead Nebula” by Jihye Hwang, Kate Pattle, Harriet Parsons, Mallory Go and Jongsoo Kim. It was published in the Astronomical Journal, an open access journal publishing original astronomical research, with an emphasis on significant scientific results derived from observations. A copy of the full scientific paper can be accessed at: https://iopscience.iop.org/article/10.3847/1538-3881/acc460
The data
The data obtained by Mallory in 2018 (with additional data obtained in 2019) was observed using POL-2, a linear polarimeter working at sub-mm wavelengths. Additional C18O spectral line data observed using the HARP instrument at the JCMT was also collected under the same program. This data was also used by the authors of this paper. The data were obtained under the Maunakea Scholar program under Directors Discretionary time for which the authors wish to thank Paul Ho.
Maunakea Scholars
The JCMT data presented in this paper was awarded under the Maunakea Scholars program. Maunakea Scholars is an innovative program designed to bring Hawaii’s aspiring young astronomers into the observatory community, competitively allocating observing time on world-class telescopes to local students. In particular the authors wish to thank Doug Simons and Mary Beth Laychak as leads of the Maunakea Scholars program.
Additional thanks
Additional thanks to Emilio Macalalad and Kapua Adolpho at Molokai High School.
JCMT
Operated by the East Asian Observatory, the James Clerk Maxwell Telescope (JCMT) is the largest astronomical telescope in the world designed specifically to operate in the submillimeter wavelength region of the spectrum. The JCMT has a diameter of 15 meters and is used to study our Solar System, interstellar and circumstellar dust and gas, and distant galaxies. It is situated near the summit of Maunakea, Hawai‘i, at an altitude of 4,092 meters.
The East Asian Observatory is a collaboration between our partner regions in China, Japan, South Korea, Taiwan, Thailand, the United Kingdom, Ireland, Canada, Hong Kong, Vietnam, Malaysia, and Indonesia.
The East Asian Observatory wishes to recognize and acknowledge the very significant cultural role and reverence that the summit of Maunakea has always had within the indigenous Hawaiian community. We are most fortunate to have the opportunity to conduct observations from this mountain.